Three battery technologies that could power the future
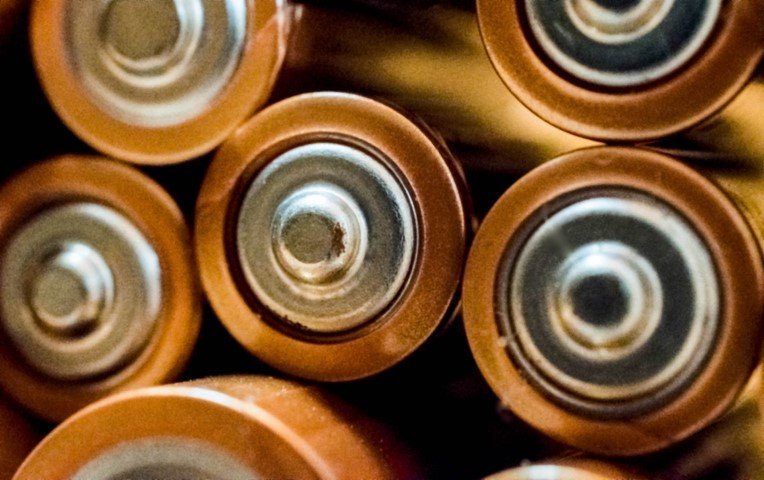
The continuum of battery technology development has been varying from stagnant periods to significant breakthroughs, in an almost unpredictable fashion. The inception of the idea about a battery charged-electric vehicle is indeed as old as the motor car itself. The trend has been consistently directing away from heavy and acid batteries to compact, light and far more efficient nickel/metal (NiMH) accumulators. One of those significant breakthroughs mentioned above came with the introduction of lithium-ion technology. Of course, many additional technological advances seem to be imminent, within the next years, through the introduction of post-lithium-ion technology.
Lithium-ion batteries are named after the movement of lithium ions within them, and they power most rechargeable devices today. The element lithium (Li) has some interesting properties that allow batteries to be both portable and powerful; the 2019 Nobel Prize in Chemistry was awarded to scientists who worked on the idea during the 1970s. But despite their widespread use, lithium-ion batteries remain extremely complicated and still intrigue scientists to unlock their secrets and open up the road for optimal efficiency.
These new batteries have also displaced the Ni-Cd (Nickel-Cadmium) ones, dominating in portable electronic devices market of smartphones and laptops. Li-ion batteries are also extensively utilized in the aerospace domain, like in the new Boeing 787, where weight and environmental-friendliness are significant factors.
Lithium-ion seems to be the most efficient battery technology available, indicating a lot of space for further improvements. They are capable of having a very high voltage and charge storage per unit mass and unit volume. They are also incomparable with the older batteries in terms of quality, output, half-life and cost. A lithium-ion (Li-ion) battery uses lithium ions as a key component of its electrochemistry. More specifically, as it goes through its discharge cycle, lithium atoms in the anode are ionized and separated from their electrons. Then, those charged lithium ions move from the anode and pass through the electrolyte until they reach the cathode, where they recombine with their electrons and practically neutralize. In principle, rechargeable batteries shouldn’t expire but they can only practically be recharged a limited number of times before they lose their ability to hold a charge. The ordinary types of battery will stop working when their terminals, the electrodes, are altered due the ions passing from one terminal of the battery to the other. In a rechargeable battery, the electrodes recover when an external charger sends those ions back where they came from.
During the last two decades, lithium-ion batteries have reached the status of being the spearhead of the automotive market. They are the same technological advancement that enabled automakers to redefine their positioning towards fossil fuels and internal combustion engines (ICE). We observe a global transition towards electric vehicles (EV), which continually pushes the boundaries of lithium-ion batteries for more power, longevity and cost-effectiveness.
For example, the ranges of 500 km are already feasible for electric vehicles, while the charging times are constantly being reduced thanks to rapid charging technology. The launch of what are known as post-lithium-ion systems are considered within-reach. New technologies, and especially the kind aimed at material-related improvements, plus ever-increasing production volumes leading to further price decreases, will determine the evolutionary development stages of the next few years. But the beauty of the battery system is not only in the cell itself and the related materials, but in the whole system that incorporates it. This includes the electronics, software, integrated cooling and the highly secure housing that is tailor-made for the vehicle and the cells.
HOW A LITHIUM ION BATTERY WORKS
The world needs more power, preferably in a form that’s clean and renewable. Our energy-storage strategies are currently shaped by lithium-ion batteries – at the cutting edge of such technology – but what can we look forward to in years to come?
Let’s begin with some battery basics. A battery is a pack of one or more cells, each of which has a positive electrode (the cathode), a negative electrode (the anode), a separator and an electrolyte. Using different chemicals and materials for these affects the properties of the battery – how much energy it can store and output, how much power it can provide or the number of times it can be discharged and recharged (also called cycling capacity).
Battery companies are constantly experimenting to find chemistries that are cheaper, denser, lighter and more powerful.
NEW GENERATION LITHIUM-ION
WHAT IS IT?
In lithium-ion (Li-ion) batteries, energy storage and release is provided by the movement of lithium ions from the positive to the negative electrode back and forth via the electrolyte. In this technology, the positive electrode acts as the initial lithium source and the negative electrode as the host for lithium. Several chemistries are gathered under the name of Li-ion batteries, as the result of decades of selection and optimization close to perfection of positive and negative active materials. Lithiated metal oxides or phosphates are the most common material used as present positive materials. Graphite, but also graphite/silicon or lithiated titanium oxides are used as negative materials.
With actual materials and cell designs, Li-ion technology is expected to reach an energy limit in the next coming years. Nevertheless, very recent discoveries of new families of disruptive active materials should unlock present limits. These innovative compounds can store more lithium in positive and negative electrodes and will allow for the first time to combine energy and power. In addition, with these new compounds, the scarcity and criticality of raw materials are also taken into account.
WHAT ARE ITS ADVANTAGES?
Today, among all the state-of-the-art storage technologies, Li-ion battery technology allows the highest level of energy density. Performances such as fast charge or temperature operating window (-50°C up to 125°C) can be fine-tuned by the large choice of cell design and chemistries. Furthermore, Li-ion batteries display additional advantages such as very low self-discharge and very long lifetime and cycling performances, typically thousands of charging/discharging cycles.
WHEN CAN WE EXPECT IT?
New generation of advanced Li-ion batteries is expected to be deployed before the first generation of solid-state batteries. They’ll be ideal for use in applications such as Energy Storage Systems for renewables and transportation (marine, railways, aviation and off road mobility) where high energy, high power and safety is mandatory.
LITHIUM-SULFUR
WHAT IS IT?
In Li-ion batteries, the lithium ions are stored in active materials acting as stable host structures during charge and discharge. In lithium-sulfur (Li-S) batteries, there are no host structures. While discharging, the lithium anode is consumed and sulfur transformed into a variety of chemical compounds; during charging, the reverse process takes place.
WHAT ARE ITS ADVANTAGES?
A Li-S battery uses very light active materials: sulfur in the positive electrode and metallic lithium as the negative electrode. This is why its theoretical energy density is extraordinarily high: four times greater than that of Li-ion. That makes it a good fit for the aviation and space industries.
Saft has selected and favoured the most promising Li-S technology based on solid state electrolyte. This technical path brings very high energy density, long life and overcomes the main drawbacks of the liquid based Li-S (limited life, high selfdischarge, …).
Furthermore, this technology is supplementary to solid state Li-ion thanks to its superior gravimetric energy density (+30% at stake in Wh/kg).
WHEN CAN WE EXPECT IT?
Major technology barriers have already been overcome and the maturity level is progressing very quickly towards full scale prototypes.
For applications requiring long battery life, this technology is expected to reach the market just after solid-state Li-ion.
SOLID-STATE
WHAT IS IT?
Solid-state batteries represent a paradigm shift in terms of technology. In modern Li-ion batteries, ions move from one electrode to another across the liquid electrolyte (also called ionic conductivity). In all-solid-state batteries, the liquid electrolyte is replaced by a solid compound which nevertheless allows lithium ions to migrate within it. This concept is far from new, but over the past 10 years – thanks to intensive worldwide research – new families of solid electrolytes have been discovered with very high ionic conductivity, similar to liquid electrolyte, allowing this particular technological barrier to be overcome.
Today, Saft R&D efforts focus on 2 main material types: polymers and inorganic compounds, aiming the synergy of the physico-chemical properties such as processability, stability, conductivity …
WHAT ARE ITS ADVANTAGES?
The first huge advantage is a marked improvement in safety at cell and battery levels: solid electrolytes are non-flammable when heated, unlike their liquid counterparts. Second, it permits the use of innovative, high-voltage high-capacity materials, enabling denser, lighter batteries with better shelf-life as a result of reduced self-discharge. Moreover, at system level, it will bring additional advantages such as simplified mechanics as well as thermal and safety management.
As the batteries can exhibit a high power-to-weight ratio, they may be ideal for use in electric vehicles.
WHEN CAN WE EXPECT IT?
Several kinds of all-solid-state batteries are likely to come to market as technological progress continues. The first will be solid-state batteries with graphite-based anodes, bringing improved energy performance and safety. In time, lighter solid-state battery technologies using a metallic lithium anode should become commercially available.
Promising battery technology
As previously stated, EV adoption is becoming a catalyst for further developments in the battery industry and vice versa. For example, an 85-kWh Lithium-ion battery of a Tesla Model S is approximately 1,200 pounds consisting of 7,104 cells. It has a great range of up to 265 miles, but it can take up to 3-4 hours to recharge at a standard 220V source.
A Chinese battery-maker, supplying most of the major automakers (including Tesla) revealed they produced the first ‘million-mile battery’. Contemporary Amperex Technology (CATL) says its new battery is capable of powering a vehicle for more than a million miles (1.2 million, to be precise – or 1.9 million km) over a 16-year lifespan.
This is why Tesla, which is today arguably considered the industry leader, is constantly reiterating and advancing on new battery technology. A new advancement is their lithium iron phosphate cathodes that are cobalt-free, something that takes care of the question the raw resource’s scarcity. Cobalt is not only scarce but also linked with cruel and unethical mining methods in developing countries around the world. Both iron and phosphorous are easy to find and mine resources and could drastically reduce the environmental impact of mining for the scarce cobalt for battery usage. They also offer batteries with longer lifecycles and higher discharge and recharge rates. The trade-off is that iron phosphate, due to lower density capacity, could force an increase in battery size. Tesla is working on finding an engineering solution, in terms of battery shape, to optimize space utilization.
As with lithium iron phosphate, Lithium-Sulphur could become another very promising approach as a replacement for heavy metals in batteries. Researchers at Monash University have been working and finally developed a Lithium-Sulphur battery design, tested on a cell phone, that held charge for five days. That could promise a lot in terms of car applications; Monash researchers theorize that Lithium-Sulphur batteries can store more energy than Lithium-ion by a factor of six. They expect to commercialize the application within the next years.
Another very promising battery technology is glass battery technology. The idea is to add sodium or even lithium to glass and form an electrode within the battery. This application could render it appropriate for mobility applications and it also seems that it’s more stable than other sources, can handle extreme temperature better and is cheaper to produce. Glass battery technology is reportedly capable of storing three times the energy of a traditional Lithium-ion battery of a similar size and can withstand many more charge and discharge cycles than typical EV batteries. This implies reducing battery size maintaining the same range and performance or maintaining the size of a vehicle and extending the range by up to three times. The 1,000-mile EV barrier could be broken eventually.
Meanwhile, Echion Technologies- a start-up hailing from England, claims they have developed an anode for high-capacity Lithium batteries to reduce drastically recharging times. The anode, which operates as a negative pole during use and a positive pole during charge, has been called a mixed niobium oxide anode. The mixed niobium oxide anode can be used in exchange for any other anode style to improve recharging. It’s compatible with conventional cathodes and electrolyte materials so it can be widely implemented. The bold claim about mixed niobium oxide anodes is that it can allow high-capacity Lithium batteries to recharge in as little as six minutes.
Applications and implications of battery technology
As already mentioned, EVs have been catalytically driving the need for improvements in battery technology and they are a segment that the new technologies will definitely shape in the future. Leading EV manufacturers like Tesla, GM, Honda, BMW, NIO, Ford and Porsche offer diverse options starting from hybrid cars, all the way to fully electric vehicles. This simply means that the battery-powered electric vehicles (BEVs) rely only on electric power to drive whereas plug-in hybrid electric vehicles (PHEVs) and full hybrid electric vehicles (FHEV) work along with internal combustion engines (ICE) to generate power for the car.
When designing EV batteries, engineers must not only consider capacity but also charging times, degradation, chemistry aspects and definitely safety. Energy and power density thresholds have been realized in most EV applications, yet vehicle manufacturers are constantly tweaking module and cell sizes for optimum performance levels. Regardless of Lithium-ion battery cell and module sizes, the high-voltage battery systems that power EVs require meticulously designed battery management systems (BMS) to ensure maximum power and safety.
Tesla, along with upstart NIO, seem to be currently leading the largest global EV market, creating vehicles with up to 110kWh battery systems. These cars can store enough energy to power a standard 60W light bulb for up to 80 days and power the Tesla Model S for 400 miles. Their most recent battery packs will pack several thousand of Tesla’s very own 2170 Lithium-ion cells. The 2170 Tesla Lithium-ion cells are 10-15% more energy efficient than the Panasonic 18650 cells at work in previous models. Tesla’s 100kWh battery solution, built around the 18650 cells, contains 8,256 cells (12Ah/cell), evenly distributed across 16 battery modules.
Meanwhile, Porsche Taycan, Porsche’s attempt at a high-performing EV, contains a 93.4kWh battery that produces 800V, instead of the standard 400V found in most other electric vehicles. The Taycan’s battery consists of 33 battery modules with 12 cells each, totalling 396 lithium-ion cells capable of storing a whopping 235.8 Wh/cell. Since battery charging speed is limited by current, the higher voltage these cells produce means lighter battery system weights and faster charging.
When it comes to hybrid solutions, Toyota is the leader. Their most popular PHEV, the Prius, boasts an 8.8 kWh battery pack, which enables the vehicle to achieve nearly 55 MPG in the city. Drivers can charge the 8.8 kWh battery at home or on the go, and because the Prius Prime consumes more electricity than gasoline, it saves money at the pump. The Prius Prime is powered by five battery stacks, each containing 19 LI cells (95 cells) that combine to a total capacity of 8.8kWh. By comparison, the standard Prius – the world’s most popular FHEV – contains a much smaller battery, with only two stacks at 28 cells each (total of 56 cells), giving it a final capacity of 0.745 kWh. Many other manufacturers offer multiple models with varying battery capacity systems and battery utilization.
Other futuristic ideas are related to Vertical Take Off and Landing (VTOL) planes, drones and many other very interesting applications. Now for the commercialization of a VTOL Taxi or, for the logistics industry to use EV’s fully and for more widespread use of electric vehicles we will need a few things, like battery longevity, increased availability of stored energy (even within our homes – with solutions like the Tesla power wall) and faster charging facilities.
Jeff Dahn, a Professor in the Department of Physics & Atmospheric Science along with his team at their Canadian lab have long been conducting leading battery research, but over the last four years, have been proceeding in partnership with Tesla. They have also been looking at new battery technology that does not just slightly improve batteries but changes them completely. But Dahn and Tesla’s research shows a very different path – Anode free, Lithium pouch cells with dual-salt LiDFOB/LiBF4 Liquid Electrolyte. Professor Dahn, along with Tesla’s scientific team, stated in one of their papers: “Recently, we demonstrated long-lifetime anode-free cells using a dual-salt carbonate electrolyte. Here we characterize the degradation of anode-free cells with this lean (2.6 g AG-1) Liquid electrolyte. We observe deterioration of the pristine Lithium morphology using scanning electron microscopy and X-ray tomography and diagnose the cause as electrolyte degradation and depletion using nuclear magnetic resonance spectroscopy and ultrasonic transmission mapping. For the safety characterization tests, we measure the cell temperature during nail penetration.”
If Tesla keeps pushing in this way, battery cell efficiency will go through the roof, meaning that not only will we have high quality cells available to us, but we will also then be able to properly utilize power wall(s) in our homes, our work and why not in public places. In theory, this would mean that we could store our own solar energy better, or in the case of commercialization, energy companies could store the wind, solar and tidal energy they produce at off-peak times saving it for when demand is increased, rather than letting it go to waste. Energy costs would plummet and, as there would be public confidence in both the range and longevity of EVs, there would most likely be a significant shift in vehicular buying habits. Especially if it was combined with a governmental incentive, similar to the current German offering. When we save the energy we produce, rather than leaving it to go to waste, we would only need to use fossil fuels to top up our energy demands, rather than using it to provide the bulk of them.